The Digital HFC — A Path to 10G
By Steven Harris
Seasoned telecommunication professionals are well aware of the improvements digital video offered over analog video in the network. Operators leveraged Moving Picture Experts Group (MPEG) digital video and RF quadrature amplitude modulation (QAM) to transport multiple standard definition (SD), high definition (HD) and ultra HD (UHD) channels in the same amount of RF spectrum that was previously used for analog video. The was not only a massive improvement in capacity, but an enhancement in quality, impacting the customer experience in a positive manner. Our industry is always on the hunt for areas of innovation in the network. Today, one such innovation is the use of digital fiber connectivity instead of analog fiber connectivity in the network.
In our access networks, fiber connectivity was first used to boost the tree and branch architecture. Fiber replaced the coaxial trunk in the tree and branch network, reducing distortions, noise and cascade depth, creating an improved architecture called the hybrid fiber/coax (HFC) network. The optical connectivity used to create HFC used an analog signal for communication, called analog intensity modulation (AIM), which this article will refer to as analog fiber. Analog fiber interfaced well with existing telecommunication equipment. The analog fiber transmitters (Tx) and analog fiber receivers (Rx) were able to use existing RF signals, directly applying Rx signals to the coaxial cable portion of the network without further signal processing. However, analog fiber requires relatively high optical output power at the hubsite Tx, a high degree of linearity, and typically only operates over shorter optical links. A typical analog fiber Tx produces roughly +6 dBm while an analog fiber Rx (e.g., optical node) requires an input of 0 dBm to –3 dBm. The amplitude of the RF signal or electrical signal at the output of the analog fiber link depends on the amount of optical power at the input of the optical Rx.
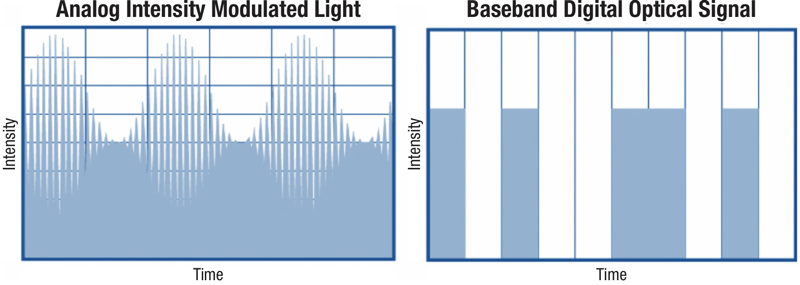
An alternative to analog fiber is baseband digital modulation, which we will refer to a digital fiber onward. In digital fiber, optical pulses of light are transformed into binary 0s and 1s. A simple way to explain its operation, would be that a non-active laser pulse generates a 0, while an active laser pulse generates a 1. To form the optical signal and to transmit digital data over fiber optics, the laser must be turned on and turned off millions or billions of times per second. Access networks that use digital fiber from the hubsite to the node may be referred to as a digital HFC (DHFC). Digital fiber requires less optical power at the hubsite Tx, a lower degree of linearity, and typically operates over longer optical links using Ethernet via 10 Gbps SFPs. A typical 10 gigabit (Gbps) digital fiber Tx produces roughly +1 dBm to -4 dBm, while a 10 Gbps digital fiber Rx (e.g., RPD) requires an input of -4 dBm to –24 dBm, depending on the optical power budget and vendor used. At the Tx, the information must be sent via 0s and 1s instead of as an RF signal, and within the Rx, an RF signal must be created from the 0s and 1s.
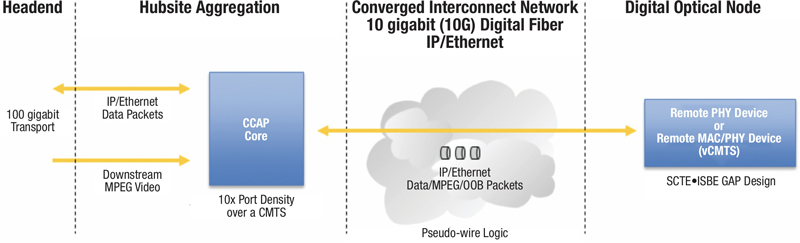
As operators enable an all-digital future, digital fiber will more cost-effectively and more reliably deliver gigabit speeds to customers and will scale to the 10 Gbps and future 100 Gbps networks needed by our industry. This is the reason the industry has defined a broadband technology platform called 10G. The platform addresses issues with increasing symmetrical speeds, driving lower latencies, enhancing reliability, delivering higher compute capabilities and providing better security in a scalable manner. Visit the CableLabs® 10G portal or the SCTE·ISBE MicroLessons portal for more information, as the reminder of the article will focus on the benefits of digital fiber.
The majority of telecommunication operators aligned their roadmaps to the 10G goals, transforming the access network and extending fiber deeper. Digital fiber, along with improved compute power will further the development of the converged interconnect network (CIN). The CIN is a key element of the distributed access architecture (DAA), providing digital fiber deep into the access network, closer to the customer premises. The CIN provides the necessary IP routing and Ethernet switching functions. In addition, the CIN connects an operator’s hubsite to a digital access network’s physical device (e.g., remote PHY device) or to an access network’s media access control device (e.g., remote MAC/PHY device).
Deep digital fiber improves telecommunication signaling in the forward path as well as the return path. Fiber’s strength is that optics offers a lower signal loss per unit length than coaxial cable. A reduction of coax and an increase in digital fiber in the access network reduces signal loss, offers lower operational expenses (OpEx), reduces distortions, reduces noise, expands dynamic range, and strengthens RF signal metrics (e.g., MER or BER) in the coaxial portion of the network. By strengthening RF signal metrics over the coax network, operators have the ability to achieve higher modulation orders (e.g., 4096-QAM) and leverage advanced multiplexing (e.g., orthogonal frequency division multiplexing) with better reliability. In addition, digital fiber with dense wavelength division multiplexing (DWDM) providing scaling at the CCAP port, with vendors sharing up to 10x the port density over a traditional CMTS. Digital fiber connections are able to adapt as the network evolves, using more wavelengths per fiber, and leveraging denser DWDM grids. Due to the higher densification of nodes and 10+ Gbps connections from existing CCAP equipment, a hubsite will need to aggregate an increased amount of traffic. A high-capacity 100 Gbps digital fiber transport between the hubsite and the headend is required to handle future capacity. These digital fiber networks provide a clear path for future FTTx deployments like Ethernet passive optical network (EPON), leveraging SCTE·ISBE standards projects like the generic access platform (GAP).
Distributed architectures that leverage digital fiber set the stage for future virtualization (e.g., vCCAP) of headend functions to provide scalability, agility, greater bandwidth, better customer experiences, secure environments and reduced operational complexity. All the pillars of the 10G platform address future networks, where these platforms will be 10x faster than they are today. In addition to 10G, the IEEE has developed standards for 25 Gbps Ethernet (802.3by) and 50 Gbps Ethernet (802.3cd) to grow networks with even greater bandwidth.
10G digital fiber networks set the stage for new services in the telecommunication network. Operators will be able to provide transport and access for 5G towers and 5G new radio (NR) base stations. Digital fiber offers a boundless foundation for DOCSIS 4.0 full duplex (FDX) DOCSIS and extended spectrum DOCSIS (ESD), improving the growth of high-speed Internet (HSI). Digital fiber sets the foundation for new video service or improving existing video services: Internet protocol television (IPTV), 4k high dynamic range (HDR), 8k HDR, virtual reality (VR) and augmented reality (AR). As mentioned, digital fiber is a launching point for FTTH/FTTx, and even DWDM coherent optics that will play a central role in our networks in the near future. Coherent optics allow for multiple bits to be transmitted in digital fiber, using QAM over digital fiber.
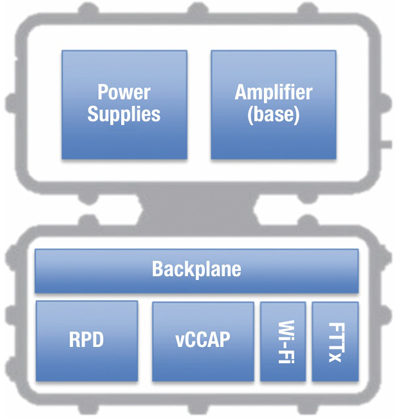
As we advanced our industry and customer experiences, the dependence on technology is expediential and critical to people’s lives. The SCTE·ISBE has been leading, creating and stepping up to support the industry in the 10G movement. Be sure to keep up to date in our industry; join the SCTE·ISBE as a member or consider earning an industry certification. If you are interested in developing new skills for the future network, check out all the new courses, like DAA Essentials.
Steven Harris
Executive Director, Technical Sales, Learning & Development
SCTE•ISBE
sharris@scte.org
Steve is an international SME and thought leader, and the executive director of education and business development for SCTE·ISBE. He is responsible for overseeing the architecture and evolution of educational programs, credentialing, and customized career progressions, as well as business development and partnerships. His team is responsible for an education library that is now 900+ modules, designed to drive business results. With more than 30 years in education, he has taught much of the content of the library, with a dynamic approach to the delivery of highly complex topics.